Ion exchange chromatography in monoclonal antibodies purification
Analytics, Manufacturing
- Ion-exchange chromatography (IEX) is a commonly used method for process-scale purification of therapeutic proteins, including monoclonal antibodies. Two main types of IEX are distinguished: anion- and cation-exchange chromatography (AEX and CEX, respectively). In AEX, negatively charged molecules bind to a positively charged stationary phase, whereas in CEX positively charged molecules interact with a negatively charged stationary phase.
- Most human antibodies have a high isoelectric point (pI), which means they generally do not bind to AEX resins under typical pH conditions. Consequently, AEX is often used in flow-through mode, allowing antibodies to pass through while capturing negatively charged impurities on the resin. In contrast, CEX is highly effective at removing host cell proteins (HCPs), leached Protein A, and aggregates.
- The experimental setup of IEX is primarily influenced by the pI of the tested protein, choice of stationary phase, composition of the mobile phase and elution method. Salt-gradient or pH-gradient elution mode, or combination of both can be used to achieve optimal separation.
Background
Ion exchange chromatography (IEX) is a separation technique used for polar and charged molecules, based on their ion exchange interactions with an oppositely charged stationary phase. The application of IEX in scientific research dates back to 1958, when Moore, Stein and Spackman successfully separated 22 amino acids using sulphonated polystyrene resins.1 Since the 1980s, ion exchange chromatography has become a fundamental part of physicochemical separation methodologies, particularly after the development of detection methods for small ions resolved on ion exchange resins.2
The mechanism of ion exchange separation is based on the competition between the ions in the sample and the charged components of eluent phase for binding to inversely charged functional groups in the stationary phase. The selectivity of IEX method is influenced by several factors, including stationary and mobile phase characteristics, type of solute ions, and secondary factors such as hydrophobic interactions and solvation effects.3
IEX can be categorized into two main modes of separation, based on the charge of ion exchanger within the stationary phase. In anion exchange chromatography (AEX), negatively charged molecules interact with a positively charged stationary phase. Conversely, in cation exchange chromatography (CEX), positively charged molecules bind to a negatively charged stationary phase.4
The key advantages of IEX, recognized over the years, such as versatility of application, relatively low cost and high-throughput possibilities, enabled this technique to be used for purification and study of peptides, proteins (i.e. antibodies), enzymes as well as organic chemicals.5-8 A fundamental application of ion exchange chromatography in biomolecule purification relies on their ampholytic characteristics and isoelectric point (pI), which represents the pH at which molecule acquires a neutral net charge. When the pH of the mobile phase is lower than the pI of the specific biomolecule, it carries a positive net charge. Conversely, when the pH is higher, the molecule becomes negatively charged. This differential ionization of functional groups on individual biomolecules (e.g. proteins) allows for their effective separation.9
In the case of proteins, their ampholyte nature and pI are influenced by the presence of basic (lysine, arginine) and acidic (aspartate, glutamate) side chains. By adjusting mobile phase conditions, proteins of interest can be made to carry an opposite charge to that of the stationary phase, promoting efficient absorption. Elution of bounded molecules can then be accomplished by altering the mobile phase pH to change the net charge of the absorbed proteins. More commonly used is the increase of the concentration of charged components in the mobile phase that competes with bound proteins as elution factor. Based on the aforementioned principles, These principles have made IEX a staple in pharmaceutical industries for both preparative and analytical applications, particularly in the purification and physicochemical characterization of proteins.
Ion-exchange chromatography in protein purification
In the biopharmaceutical industry, IEX chromatography is commonly used for process-scale purification of monoclonal antibodies (mAbs), fusion proteins and other protein-based therapeutics. IEX is the most well-characterized chromatography method, which along with wide availability of various ion exchange resins, allows for tailored processes that maximize purification efficiency. For mAbs purification, ion exchange chromatography plays a major role as a method of eliminating both process- and product-related impurities, ensuring the high quality of the final product.10
Anion-exchange chromatography in antibodies’ purification
AEX chromatography is frequently used as a polishing step in antibodies purification process, following a capture step via Protein A affinity chromatography. Because the high isoelectric point (pI) of most human antibodies prevents them from binding to AEX resins at typical pH conditions (pH 7.0–8.0), AEX is often run in a flow-through mode, allowing antibodies to pass through while retaining impurities on the resin. While higher pH levels can enhance antibody binding, they are generally avoided to reduce the risk of deamidation and proteolysis.11
In contrast, impurities such as DNA, host cell proteins (HCPs), and endotoxins are negatively charged and bind strongly to AEX columns under the same conditions. AEX in flow-through mode also offers excellent virus removal and is validated as a reliable method for virus clearance.12 However, it is less effective at removing aggregates and leached Protein A, which are typically addressed in subsequent polishing steps. Since contaminants remaining after Protein A affinity chromatography are typically in trace amounts (ppm or ppb), AEX flow-through step can be performed at high loading capacities of up to 100 mg/mL of resin.10
Table 1 lists some of the commonly used resins for this chromatography method. Membrane chromatography is a viable alternative to AEX chromatography in flow-through mode. Although membrane chromatography has a lower binding capacity due to a reduced surface area-to-bed volume ratio, this limitation is not an issue in flow-through mode, where only trace contaminants need to bind. Examples of AEX chromatography membranes available on the market are provided in Table 2.
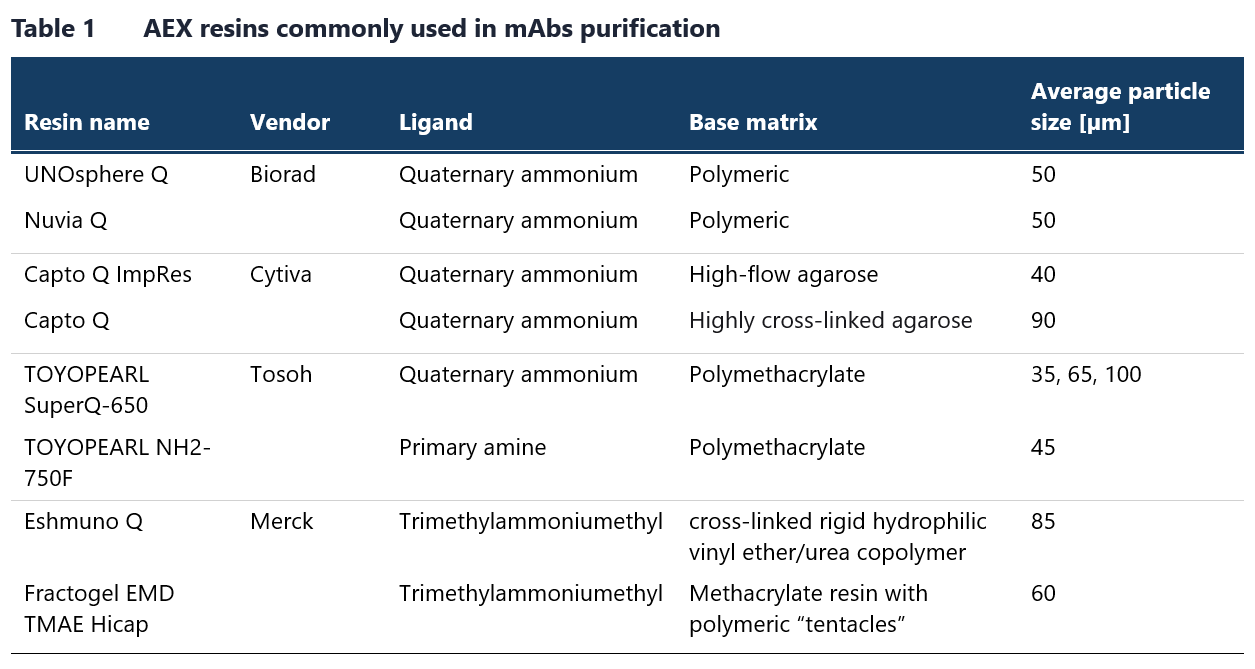
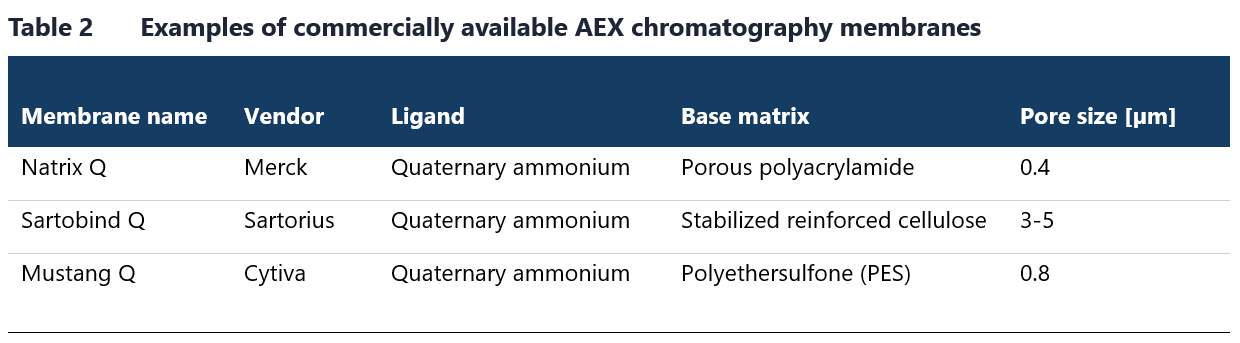
The AEX step can also be executed in an isocratic mode known as weak-partitioning chromatography (WPC).13 In this mode, conditions are optimized to promote substantial product binding, typically in the range of 1–20 g/L of resin). Since antibodies are generally more basic than many impurities, the latter bind more strongly to the resin, facilitating separation through sample displacement. By using elevated loading capacities (up to 250 g/L of resin) and brief isocratic washes, high product yields of >95% can be achieved. Operating the AEX step in this WPC mode preserves the isocratic conditions typical of AEX flow-through steps while enhancing the selectivity for impurity removal. When used as the sole polishing step, WPC has demonstrated significant impurity clearance, with log reduction values (LRV) of approximately 4 for HPCs, over 2 for leached Protein A, over 3 for nucleic acids, over 5 for retroviruses, and ~ 20-fold reduction in product aggregates.14 It is also advisable to test AEX in both binding and flow-through modes, as the surface charge distribution of antibody may allow for effective binding under the suitable solution conditions.
Cation-exchange chromatography in antibodies’ purification
CEX chromatography is typically employed as another polishing step in antibody purification. Given the high pI of most human antibodies, this process is usually run in bind-elute mode, which allows for high loading capacities and good selectivity. CEX is particularly effective in removing HCPs, leached Protein A, and, in some cases, high-molecular-weight (HMW) aggregates.15 It is especially efficient at reducing the levels of leached Protein A, as this protein is relatively acidic and binds less strongly to the resin than antibodies, making it easier to remove during flow-through or intermediate pH wash. Fragments of leached Protein A that bound to antibodies can be also eliminated with an intermediate pH wash.10
Table 3 lists strong CEX resins commonly used in antibody manufacturing. Although weak cation exchangers like carboxymethyl resins are used less frequently, they are also available from the listed vendors. Resins with a polymeric backbone, such as methacrylate, demonstrate enhanced selectivity for aggregate removal due to nonspecific hydrophobic interactions with the backbone.16
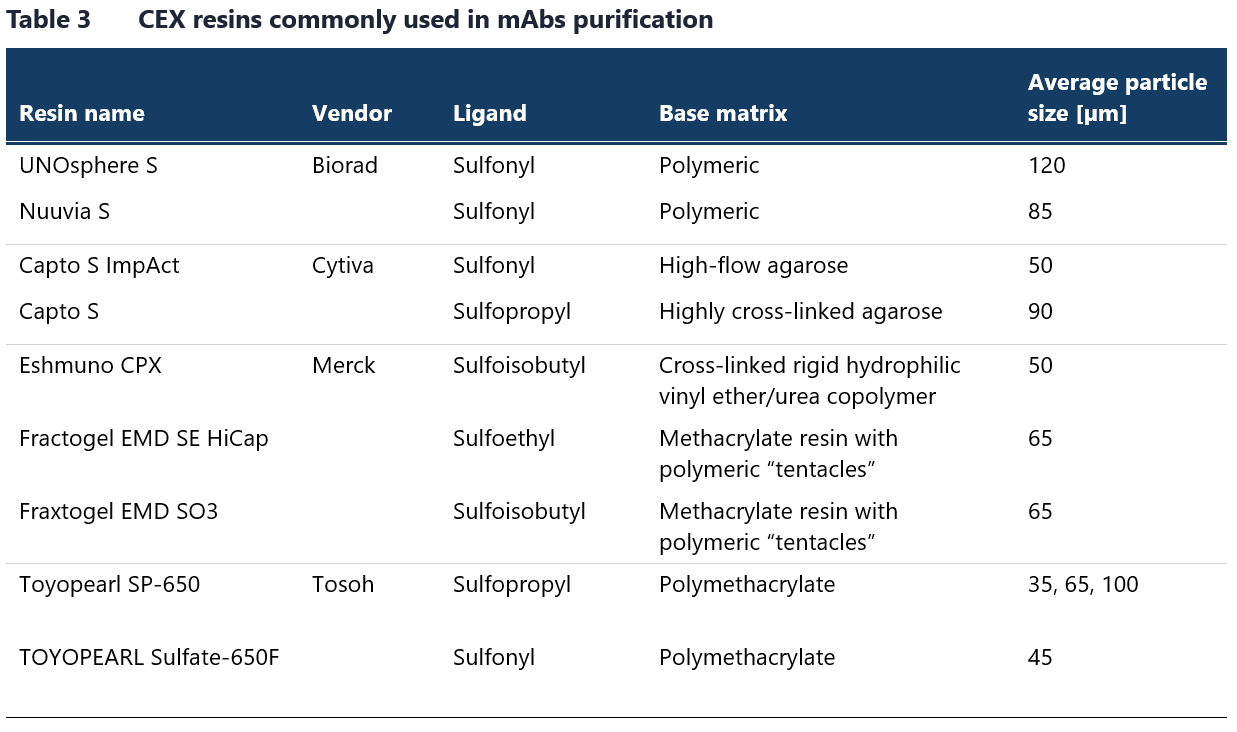
The choice of CEX resin and the optimization of operating conditions are primarily determined by the nature of the impurities to be removed and the specific antibody characteristics. While most antibodies are positively charged under standard CEX conditions, their retention on CEX resins can vary considerably. Key factors in resin selection include binding capacity and selectivity. CEX resins can provide high binding capacities for mAbs, reaching up to 100 mg/mL.17 Typically, static binding capacity is measured in a high-throughput format across multiple loading conditions (varying pH and conductivity), which helps predict dynamic binding capacity trends under selected conditions. While it is generally expected that maximum CEX capacity occurs at lower conductivity and acidic pH – conditions that result in antibodies becoming more protonated – binding capacity can sometimes increase with rising conductivity and decreasing protein charge at lower ionic strength.18
This unexpected behavior is attributed to an exclusion mechanism, where antibodies can bind to the outer pore regions of the resin and electrostatically hinder subsequent molecules from entering. As ionic strength and pH increase, the protein charges become shielded, reducing this exclusion effect andleading to higher binding capacity. However, when pH and conductivity continue to rise, the traditional trend of decreased capacity resumes, as the interactions between the resin and antibodies diminish. This observation is crucial and should be considered during process development as optimizing binding conditions can significantly impact the efficiency of the purification process.10
Scalable approaches in IEX chromatography
IEX chromatography can be performed in various ways depending on the scale and specific requirements of the application. For small-scale work, the method choice should consider the available equipment, purification purpose and desired purity. High-throughput process development (HTPD), for example, benefits from tools such as resin-based 96-well plates. When exceptionally high resolution is needed for purification of mAb charge variants, semi-preparative weak cation exchange (WCX) HPLC columns can be used. An example of preparative, high-resolution separation is shown in Figure 1.
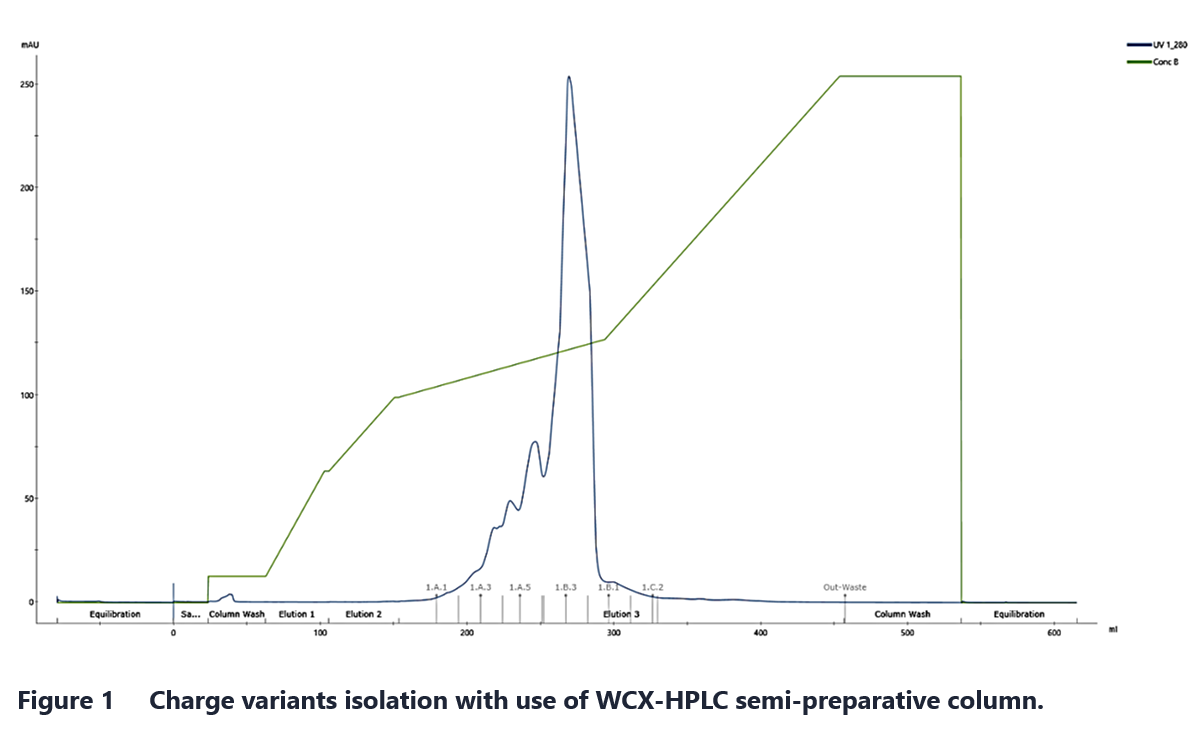
For large-scale process applications, where consistent, highly purified antibody production across multiple batches is required, large automated systems are employed. These systems, coupled with large columns filled with chromatography media or membranes, allow for effective separation across varying flow rates and capacities.
mAb purification often relies on standardized platform processes, which streamline development, reduce time-to-market and minimize early-stage material usage. Given the increasing number of mAbs in development for biopharmaceutical and bioanalytical applications, there is a growing need for optimized and scalable processes that surpass predefined sequences and materials. One promising approach for achieving this is through in silico methods in process development. Recent advancements in numerical methods now allow for the development of complex mechanistic models that accurately describe mass transfer at the bead level within chromatography resins. These models can significantly enhance the performance of separation steps in IEX chromatography.
Developing a mechanistic model involves three primary steps: modeling mass transfer, modeling binding, and validating the model.19 The level of detail in these models is determined by the desired predictive accuracy. These models require the determination of specific parameters for both protein and the absorbent, meaning they cannot be used to predict the behavior of new molecules ab initio. However, for well-characterized mAbs and resins, various modeling techniques can effectively assess the impact of factors such as mobile-phase composition, resin type, and protein characteristics on IEX chromatography performance.20
Isoform isolation
An important aspect of charge variant analysis is assessing how charge heterogeneity impacts biological activity. To isolate charge variants and assess their biological effects, semipreparative cation exchange chromatography is commonly employed. This approach enables the isolation of individual fractions, which can then be subjected to biological assays to determine the extent to which charge heterogeneity influences biological functions.21
At Mabion, we utilize semi-preparative liquid chromatography systems that facilitate the isolation of isoforms for structural characterization and evaluation of the biological activity of individual fractions. Structural analysis can be conducted using a UHPLC-MS (Q-Orbitrap) system, which enables direct analysis of charge variants via IEX with MS-compatible mobile phases. The UHPLC system also supports 2D analyses using two different chromatographic modes and allows for fraction collection and subsequent MS identification.
Analytical aspects of ion-exchange chromatography
Therapeutic proteins, particularly monoclonal antibodies (mAbs) and their derivatives, represent a dynamically growing branch of the pharmaceutical industry with ever-increasing number of innovative molecules entering the market. Thorough physicochemical characterization of the candidate drugs is essential to ensure their efficacy and safety before reaching the patients. One of the key attributes examined in mAbs is charge heterogeneity. Charge variations on peptide chains may influence the structure, stability, binding affinity and biological activity of monoclonal antibodies.
Several conditions can introduce significant heterogeneity in the charge properties of monoclonal antibodies. Even minor structure alterations can change the local distribution of charged residues or modify their pKa value, thereby affecting the overall surface-charge distribution of the antibody. For example, oxidation may bring about unintended conformational changes and increase the propensity to form aggregates, which in turn could result in increased size and charge modifications.
Additionally, several biochemical processes and post-translational modifications, such as C-terminal lysine truncation or deamidation can cause a shift in isoelectric point of an antibody resulting in the production of several charge variants.22 Table 4 lists the main modifications that affect the charge heterogeneity of therapeutic mAbs.
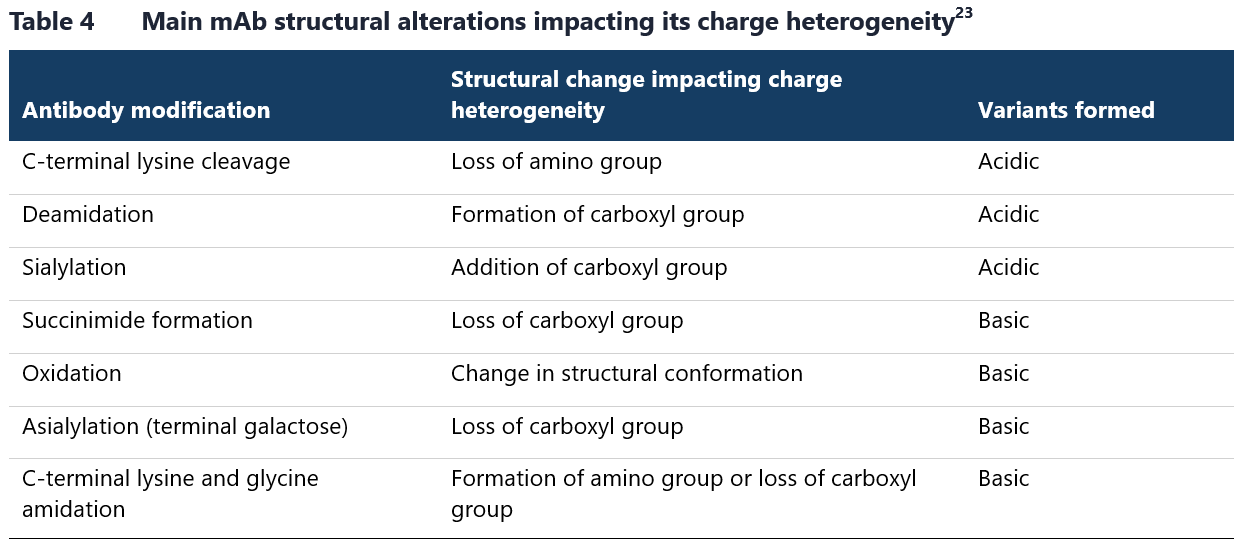
Ion exchange chromatography is one of the most widely utilized techniques for assessing charge heterogeneity in analytical applications. The implementation of HPLC and UHPLC equipment for IEX analytical methods provides sufficient separation quality of complex charge variant samples while ensuring short analysis times and operational simplicity. Nowadays, IEX is regarded as the reference method for both quantitative and qualitative assessment of charge heterogeneity in therapeutic proteins, including monoclonal antibodies.24
Key design issues in IEX analysis
The experimental setup for IEX chromatography is influenced by several factors including pI of the tested protein, stationary phase characteristics, mobile phase composition, chosen elution mode and detection method for the eluted material.
The isoelectric point of separated protein is arguably the most critical factor determining the IEX separation process. As mentioned earlier, pI determines the net charge of protein molecules under specific pH conditions, enabling their separation. These conditions should be carefully considered as proteins are usually stable only within the narrow pH range. A protein stable at pH below pI will carry a net positive charge, while at pH above its pI, it will acquire a net negative charge. These properties directly determine the choice of stationary phase – whether to use a cation or anion exchanger. Furthermore, it also impacts the selection of the starting mobile phase, which to obtain optimal separation is recommended to be at least one pH unit below (in CEX) or above (in AEX) pI.25
Stationary phase itself also plays crucial role in determining IEX conditions. Ion exchangers can be divided into weak or strong, based not on their binding strength, but on their behavior across the different pH levels. A strong ion exchanger maintains a relatively constant charge across a wider operational pH range, whereas a weak ion exchanger’s charge may fluctuate significantly with changes in pH. The characteristics of both cation and anion exchangers are defined by functional groups immobilized on the resins.26 The most common ionized chemical groups used in IEX analyses are listed in Table 5.
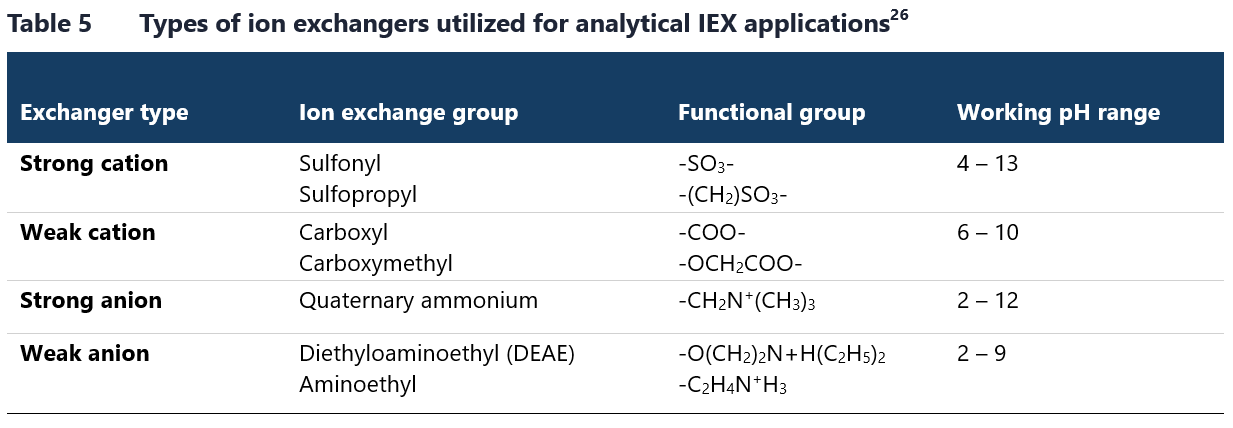
The utilized mobile phases are closely associated with the applied elution mode. In ion exchange chromatography, it is feasible to adopt either a salt-gradient or pH-gradient elution mode, or combination of both. The mobile phase should be composed of buffering ions that do not interact with the ion-exchanger groups and should have an appropriate buffering capacity, which typically requires a buffer concentration of at least 10 mM.9 For salt-gradient approach, the pH of the mobile phase should be between the pI of the analyzed protein and the pKa of functional groups immobilized within the stationary phase. The protein’s stability at the selected pH of binding buffer should be also taken into consideration. The most frequently used buffer components are citrate, phosphate and MES.27
The elution process is optimized by picking appropriate ionic strength and its gradient. Sodium chloride or potassium chloride is most commonly used as the salt, with gradient ranging from 0 to 500 mM. In the pH-gradient mode, the ionic strength of the mobile phase remains constant, while the pH during elution is gradually adjusted to reduce the protein’s interactions with the stationary phase. Weak acid combinations are typically used for low pH ranges, while buffers with amine components are used for high pH ranges.28 Imidazole, piperazine and Tris are among the most frequently employed components of the mobile phase.28
After IEX separation, the eluted charge variants are commonly detected with the use of UV absorbance method with diode array detectors (DAD) operating at 280 nm. This method takes advantage of the established light-absorbing properties of aromatic amino acid residues of protein molecules. Recently, fluorescence-based detection is also gaining importance as fluorescence light detectors (FLD) offer higher sensitivity for proteins rich in aromatic amino acids. Moreover, it is a viable alternative method for detecting the analytes with poor UV absorbance.30
IEX analytical applications
One of the most important applications of IEX within the pharmaceutical industry is its use in characterizing charge heterogeneity of monoclonal antibodies. Manufacturing and storage conditions can introduce significant changes to the charge properties of mAbs. As mentioned earlier, charge profile modifications can greatly influence the structural integrity and biological functions of a therapeutic mAb, and in consequence alter its pharmacologic profile in vivo. It follows then that the shifts in isoelectric point can have far-reaching effects on the clinical performance of the affected drug product.22 There are numerous examples of how IEX is applied to study the charge heterogeneity of mAbs including analyses of oxidized molecules30, products of antibody degradation32 and samples exposed to stress conditions.29 As a company with first-hand experience in biologics manufacturing and characterization, Mabion offers a range of in-house chromatographic methods for charge profiling studies including WCX-HPLC and SCX-UHPLC.
Representative chromatograms from WCX separation of rituximab and SCX analysis of cetuximab are illustrated in Figure 2 and Figure 3, respectively.
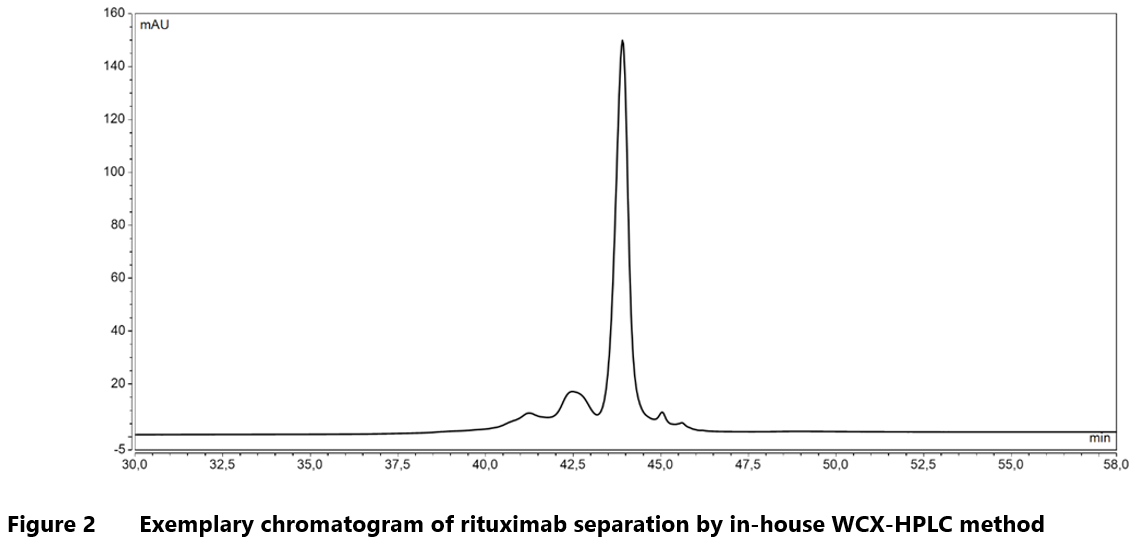
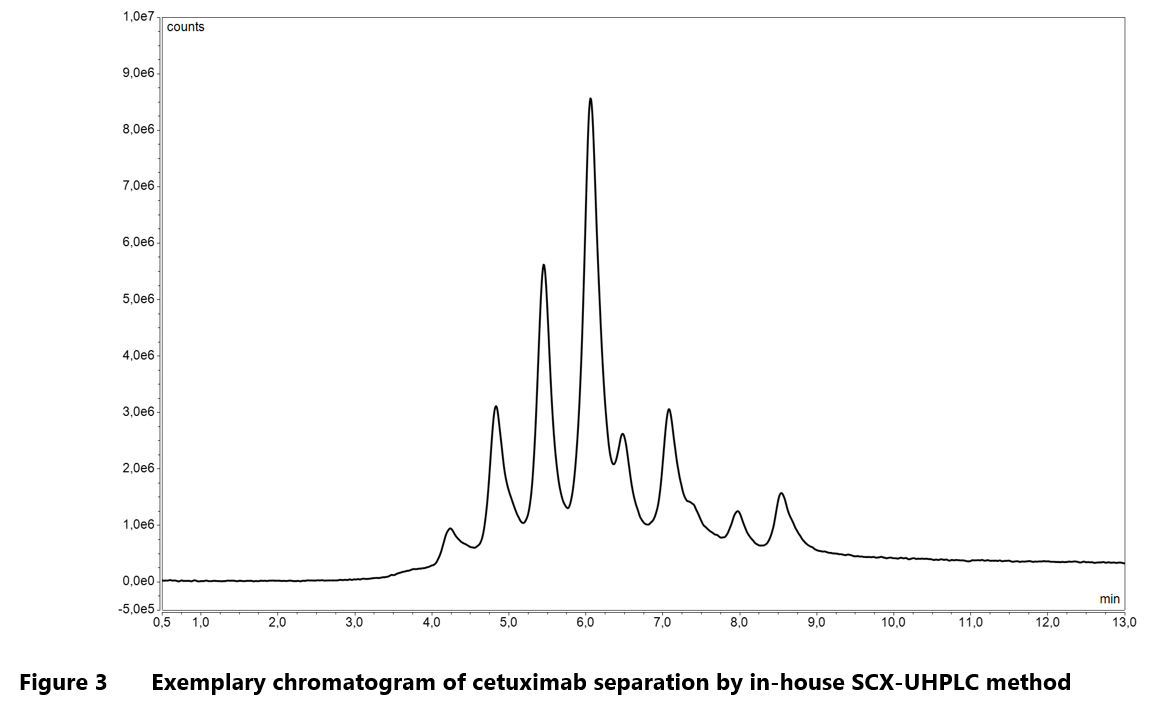
Mabion’s in-house methods for charge heterogeneity analysis utilize salt-gradient as well as combined, salt-mediated pH-gradient procedure for elution step. This flexibility enhances the analytical capabilities, enabling characterization of a broad spectrum of monoclonal antibodies.
In addition to standard monoclonals, ion chromatography exchange techniques are increasingly used for characterizing more innovative biotherapeutic molecules such as bispecific antibodies (bsAbs) or antibody-drug conjugates (ADCs).
Bispecifics are engineered antibodies that bind two different antigens or epitopes. They typically incorporate two antigen-binding domains for simultaneous interaction with distinct target cells or molecules.32 One of the key challenges in bsAbs manufacturing is the formation of mispaired species, where light or heavy chains are incorrectly paired, compromising the molecule’s bispecific functions. In addition to recognizing modifications such as deamidation, oxidation or glycation, CEX can be effectively used to analyze these mispaired species by separating heterodimers and homodimers of bispecific antibody chains.34
Antibody-drug conjugates are composed of an antibody linked to a cytotoxic agent via a chemical linker. ADCs are regarded as a form vectorized chemotherapy that allows targeted delivery of a potent drug to the specific sites, typically cancer cells.35 The main quality attribute of ADCs is the drug-to-antibody ratio (DAR), which refers to the average number of drug molecules attached to a single antibody. Depending on the conjugation site, incorporation of cytotoxic agent can alter the physicochemical properties of antibody. In cases where the drug is conjugated via lysine residues, successive attachment of payloads leads to decrease in pI, shifting the charge profile toward the acidic side. Therefore, for this type of ADCs, IEX method can be used to quantify the efficiency of drug conjugation.36 IEX-based methods are also widely utilized to study charge profile of other proteins, highlighting their versatility. The application of CEX to charge variant analysis of such molecules as cytochrome C, granulocyte colony stimulating factor (G-CSF) or ribonuclease B is well described in the literature.37-39
Innovative approach to IEX analysis
An increasing trend has emerged over the years to overstep the limitations of traditional IEX methods, particularly their insufficient resolving power or lack of compatibility with mass spectrometry. This shift has been caused by a growing demand for methods capable of analyzing increasingly complex samples such as ADCs or multispecific antibodies. Most promising solutions include multidimensional chromatographic techniques (especially two-dimensional or 2D-LC), which are gaining widespread use in the pharmaceutical industry for characterizing highly complex biomolecules. As the name suggests, two-dimensional chromatography involves the sequential application of two different separation methods. After the initial separation in the first dimension, fractions are further resolved on a second column with different selectivity. This approach enables analysis based on two distinct physicochemical properties, thereby enhancing resolution and providing more detailed information about the tested molecules.40
This technique is also being applied to IEX methods. A commonly used combination in 2D-LC is ion exchange chromatography coupled with reversed-phase liquid chromatography (IEX-RPLC). This setup allows for the separation of substances based on both charge and hydrophobicity, while also ensuring compatibility with mass spectrometry (MS). An excellent example of IEX-RPLC implementation is its use in the simultaneous analysis of DAR and payload distribution in ADCs.41
Additionally, capillary IEX methods have been explored as a way to enhance the sensitivity of these techniques while reducing sample consumption. This approach has also been successfully adapted to a two-dimensional format, enabling the effective separation of standard protein samples.42 These advancements in multidimensional chromatography represent significant progress in the ability to analyze complex biomolecules with greater precision and efficiency.
IEX for comprehensive structural and functional analysis of charge variants
After ion exchange (IEX) separation, detection is typically performed using absorbance or fluorescence detectors, which provide a signal that enables the identification of acidic and basic isoforms, as well as the so-called “main peak.” However, to fully understand the structural basis of charge heterogeneity and its impact on biological functions, a more detailed analysis is required to identify the specific modifications responsible for each fraction and their influence on biological activity. Several advanced approaches can facilitate this level of characterization.
One of such approaches is enzymatic digestion, which improves the separation of individual protein fractions, including subfractions, allowing for a more precise analysis. While resolving charge variants in small proteins is relatively straightforward, larger molecules like antibodies present a greater challenge due to their complexity and the greater number of potential charge variants. Enzymatic digestion prior to IEX separation generates different protein fragments, simplifying the separation process and enabling more accurate identification of modifications within specific regions of the protein.
For example, in a study by K.G. Moorhouse et al., the enzyme papain was used to cleave antibodies into Fab and Fc regions before chromatographic analysis. This allowed for the resolution of variants caused by glutamine cyclization to pyroglutamate at the N-terminus of both light and heavy chains (Fab-pE/Q variants), as well as variants related to the removal of C-terminal lysine from none, one, or both heavy chains (Fc-Lys variants).43 Similarly, in a study by Leblanc et al., the enzyme IdeS was used to cleave antibodies at the hinge region, producing Fc/2 and F(ab’)2 fragments. This enabled the differentiation between non-deamidated forms and those with one or two deamidations in the dimeric Fc/2 form.44 The enzymatic approach also allows for targeted analysis of specific modifications, such as the use of carboxypeptidase B to identify C-terminal lysine variants.45
The primary technique for detailed analysis of modifications responsible for specific fractions is mass spectrometry. However, IEX traditionally uses non-volatile salts, which are incompatible with direct coupling to MS. To overcome this, several strategies have been developed. One method is to collect the IEX-separated fractions and then analyze them using MS. Another technique involves two-dimensional liquid chromatography coupled with mass spectrometry (2D-LC–MS), where a second chromatographic dimension uses MS-compatible mobile phases, allowing for the assignment of peaks from the first IEX separation. Alternatively, volatile salts, such as ammonium formate or ammonium acetate, can be used in IEX to maintain compatibility with MS. This approach can be combined with enzymatic digestion to further enhance the separation and enable more accurate mass determination. This is particularly important for identifying modifications that result in small mass differences, such as deamidation.44 These advanced techniques offer greater precision in characterizing charge variants and understanding their effects on therapeutic proteins.
Prepared by:
Anna Morawska
Piotr Miłek
Małgorzata Urbaniak
References
- Moore S, Stein WH. Chromatography of amino acids on sulfonated polystyrene resins. J Biol Chem. 1951 Oct;192(2):663-81.
- Small H, Stevens TS, and Bauman WC. Novel ion exchange chromatographic method using conductimetric detection. Anal. Chem. 1975;47(11):1801-9. doi:10.1021/ac60361a017.
- Paull B, Nesterenko PN. Chapter 8 – Ion Chromatography. In: Fanali S, Haddad PR, Poole CF, Schoenmakers P, Lloyd D, (eds.). Liquid Chromatography, Elsevier, 2013, pp. 157-91, ISBN 9780124158078, https://doi.org/10.1016/B978-0-12-415807-8.00008-0.
- Fritz JS, Gjerde DT. Ion Chromatography (Fourth Completely Revised and Enlarged Edition). Weinhein: Wiley-VCH Verlag GmbH & KGoA Weinhein; 2009.
- Mant CT, Hodges RS. Mixed-mode hydrophilic interaction/cation-exchange chromatography: separation of complex mixtures of peptides of varying charge and hydrophobicity. J Sep Sci. 2008 May;31(9):1573-84. doi: 10.1002/jssc.200700619.
- Kent UM. Purification of antibodies using ion-exchange chromatography. Methods Mol Biol. 1999;115:19-22. doi: 10.1385/1-59259-213-9:19. PMID: 10098160.
- Levison PR. Large-scale ion-exchange column chromatography of proteins. Comparison of different formats. J Chromatogr B Analyt Technol Biomed Life Sci. 2003 Jun 25;790(1-2):17-33. doi: 10.1016/s1570-0232(03)00087-4.
- Hajós P, Nagy L. Retention behaviors and separation of carboxylic acids by ion-exchange chromatography. J Chromatogr B Biomed Sci Appl. 1998 Oct 9;717(1-2):27-38. doi: 10.1016/s0378-4347(98)00247-3.
- Cummins PM, Rochfort KD, O’Connor BF. Ion-Exchange Chromatography: Basic Principles and Application. Methods Mol Biol. 2017;1485:209-223. doi: 10.1007/978-1-4939-6412-3_11.
- Ghose S, Jin M, Liu J, Hickey J. Chapter 7 – Integrated polishing steps for monoclonal antibody purification. In: Gottschalk U, (ed.). Process Scale Purification of Antibodies. John Wiley & Sons; 2008. doi: 10.1002/9780470444894.ch7.
- Gagnon P. Polishing methods for monoclonal IgG purification. In: Shukla AA, Etzel MR, Gadam S, (eds.). Process Scale Bioseparations for the Biopharmaceutical Industry. CRC Press; Boca Raton, FL; 2006. pp. 491-505, ISBN 9780429188985.
- Curtis S, Lee K, Blank GS, Brorson K, Xu Y. Generic/matrix evaluation of SV40 Clearance by anion exchange chromatography in flow-through mode. Biotechnol Bioeng. 2003;84:179-86.
- Kelley B, Tobler SA, Brown P, Coffman J, Godavarti R, Iskra T, Switzer M, Vunnum S. Principles of weak partitioning chromatography for anion exchange purification of monoclonal antibodies. Biotechnol Bioeng. 2007;101(3):553-66.
- Kelley B. Very large scale monoclonal antibody purification: The case for conventional unit operations. Biotechnol Prog. 2007;23:995-1008.
- Gagnon P. Purification Tools for Monoclonal Antibodies. Validated Biosystems; Tucson, AZ; 1996, ISBN 0965351599.
- Shukla AA, Han XS. Screening of chromatographic stationary phases. In: Shukla AA, Etzel MR, Gadam S, (eds.). Process Scale Bioseparations for the Biopharmaceutical Industry. CRC Press; Boca Raton, FL; 2007. pp. 227-244, ISBN 9780429188985.
- Stein A, Kiesewetter A. Cation exchange chromatography in antibody purification: pH screening for optimized binding and HCP removal. J Chromatogr B. 2007;848:151-8.
- Harinarayan C, Mueller J, Ljunglof A, Fahrner R, Van Alstine J, Van Reis R. An exclusion mechanism in ion exchange chromatography. Biotechnol Bioeng. 2006;95:775-87. doi: 10.1002/bit.21080.
- Kumar V, Rathore AS. Mechanistic modeling of preparative ion-exchange chromatography. BioPharm Int. 2017 Apr;30(4):41-5.
- Kittelmann J, Lang KMH, Ottens M, Hubbuch J. Orientation of monoclonal antibodies in ion-exchange chromatography: A predictive quantitative structure-activity relationship modeling approach. J Chromatogr A. 2017 Aug 11;1510:33-9. doi: 10.1016/j.chroma.2017.06.047.
- Yuan JJ, Gao D, Hu F, Shi Y, Wu ZH, Hu CQ, Huang XD, Fang WJ, Zhang HT, Wang HB. Isolation and characterization of charge variants of infliximab biosimilar HS626. J Chromatogr B Analyt Technol Biomed Life Sci. 2021 Jan 1;1162:122485.
- Khawli LA, Goswami S, Hutchinson R, Kwong ZW, Yang J, Wang X, Yao Z, Sreedhara A, Cano T, Tesar D, Nijem I, Allison DE, Wong PY, Kao YH, Quan C, Joshi A, Harris RJ, Motchnik P, 2010, Charge variants in IgG1: Isolation, characterization, in vitro binding properties and pharmacokinetics in rats. MAbs. 2(6):613-24. doi: 10.4161/mabs.2.6.13333.
- Majumdar R, Esfandiary R, Bishop SM, Samra HS, Middaugh CR, Volkin DB, Weis DD. Correlations between changes in conformational dynamics and physical stability in a mutant IgG1 mAb engineered for extended serum half-life. MAbs. 2015;7(1):84-95. doi: 10.4161/19420862.2014.985494.
- Fekete S, Gassner AL, Rudaz S, Schappler J, Guillarme D. Analytical strategies for the characterization of therapeutic monoclonal antibodies. Trac Trends Anal Chem. 2013;42:74–83. doi: 10.1016/j.trac.2012.09.012.
- Duong-Ly KC, Gabelli SB. Using ion exchange chromatography to purify a recombinantly expressed protein. Methods Enzymol. 2014;541:95-103. doi: 10.1016/B978-0-12-420119-4.00008-2.
- Cummins PM, Dowling O, O’Connor BF. Ion-exchange chromatography: basic principles and application to the partial purification of soluble mammalian prolyl oligopeptidase. Methods Mol Biol. 2011;681:215-28. doi: 10.1007/978-1-60761-913-0_12.
- Fekete S, Beck A, Veuthey JL, Guillarme D. Ion-exchange chromatography for the characterization of biopharmaceuticals. J Pharm Biomed Anal. 2015 Sep 10;113:43-55. doi: 10.1016/j.jpba.2015.02.037.
- Liu Y, Anderson DJ. Gradient chromatofocusing high-performance liquid chromatography. I. Practical aspects. J Chromatogr A. 1997 Feb 21;762(1-2):207-17. doi: 10.1016/s0021-9673(96)00854-0.
- Rea JC, Moreno GT, Lou Y, Farnan D. Validation of a pH gradient-based ion-exchange chromatography method for high-resolution monoclonal antibody charge variant separations. J Pharm Biomed Anal. 2011 Jan 25;54(2):317-23. doi: 10.1016/j.jpba.2010.08.030.
- Joshi S, Upadhyay K, S Rathore A. Ion exchange chromatography hyphenated with fluorescence detector as a sensitive alternative to UV detector: Applications in biopharmaceutical analysis. J Chromatogr B Analyt Technol Biomed Life Sci. 2022 Dec 1;1212:123511. doi: 10.1016/j.jchromb.2022.123511.
- Teshima G, Li MX, Danishmand R, Obi C, To R, Huang C, Kung J, Lahidji V, Freeberg J, Thorner L, Tomic M. Separation of oxidized variants of a monoclonal antibody by anion-exchange. J Chromatogr A. 2011 Apr 15;1218(15):2091-7. doi: 10.1016/j.chroma.2010.10.107.
- Lau H, Pace D, Yan B, McGrath T, Smallwood S, Patel K, Park J, Park SS, Latypov RF. Investigation of degradation processes in IgG1 monoclonal antibodies by limited proteolysis coupled with weak cation-exchange HPLC. J Chromatogr B Analyt Technol Biomed Life Sci. 2010 Apr 1;878(11-12):868-76. doi: 10.1016/j.jchromb.2010.02.003.
- Kontermann RE, Brinkmann U. Bispecific antibodies. Drug Discov Today. 2015 Jul;20(7):838-47. doi: 10.1016/j.drudis.2015.02.008.
- Duivelshof BL, Beck A, Guillarme D, D’Atri V. Bispecific antibody characterization by a combination of intact and site-specific/chain-specific LC/MS techniques, Talanta 2022;236:122836, ISSN 0039-9140.
- Joubert N, Beck A, Dumontet C, Denevault-Sabourin C. Antibody-Drug Conjugates: The Last Decade. Pharmaceuticals (Basel). 2020 Sep 14;13(9):245. doi: 10.3390/ph13090245.
- Wagh A, Song H, Zeng M, Tao L, Das TK. Challenges and new frontiers in analytical characterization of antibody-drug conjugates. MAbs. 2018 Feb/Mar;10(2):222-43. doi: 10.1080/19420862.2017.1412025.
- Gotte G, Libonati M, Laurents DV. Glycosylation and specific deamidation of ribonuclease B affect the formation of three-dimensional domain-swapped oligomers. J Biol Chem. 2003 Nov 21;278(47):46241-51. doi: 10.1074/jbc.M308470200.
- Clogston CL, Hsu YR, Boone TC, Lu HS. Detection and quantitation of recombinant granulocyte colony-stimulating factor charge isoforms: comparative analysis by cationic-exchange chromatography, isoelectric focusing gel electrophoresis, and peptide mapping. Anal Biochem. 1992 May 1;202(2):375-83. doi: 10.1016/0003-2697(92)90121-m.
- Weitzhandler M, Farnan D, Rohrer JS, Avdalovic N. Protein variant separations using cation exchange chromatography on grafted, polymeric stationary phases. Proteomics. 2001 Feb;1(2):179-85.
- Samanidou VF. Two-Dimensional Liquid Chromatography (2D-LC) for Biopharmaceuticals. Pharm Anal Acta 2018;9:e192.
- Sandra K, Vanhoenacker G, Vandenheede I, Steenbeke M, Joseph M, Sandra P. Multiple heart-cutting and comprehensive two-dimensional liquid chromatography hyphenated to mass spectrometry for the characterization of the antibody-drug conjugate ado-trastuzumab emtansine. J Chromatogr B Analyt Technol Biomed Life Sci. 2016 Oct 1;1032:119-30. doi: 10.1016/j.jchromb.2016.04.040.
- Pepaj M, Wilson SR, Novotna K, Lundanes E, Greibrokk T. Two-dimensional capillary liquid chromatography: pH gradient ion exchange and reversed phase chromatography for rapid separation of proteins. J Chromatogr A. 2006 Jul 7;1120(1-2):132-41. doi: 10.1016/j.chroma.2006.02.031.
- Moorhouse KG, Nashabeh W, Deveney J, Bjork NS, Mulkerrin MG, Ryskamp T. Validation of an HPLC method for the analysis of the charge heterogeneity of the recombinant monoclonal antibody IDEC-C2B8 after papain digestion. J Pharm Biomed Anal. 1997;16(4):593-603.
- Leblanc Y, Ramon C, Bihoreau N, Chevreux G. Charge variants characterization of a monoclonal antibody by ion exchange chromatography coupled on-line to native mass spectrometry: Case study after long-term storage at +5°C. J Chromatogr B. 2017;1048:130-9.
- Kim DG, Kim HJ, Kim HJ. Effects of carboxypeptidase B treatment and elevated temperature on recombinant monoclonal antibody charge variants in cation-exchange chromatography analysis. Arch Pharm Res. 2016;39(10):1472-81.
Related resources
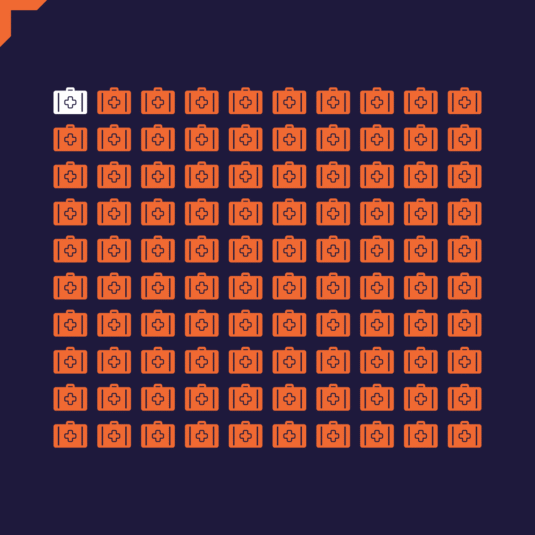
Time to Market Strategy – 5 CDMO Tactics to Speed Up Biologic Launches
Biologics, Drug development, Manufacturing
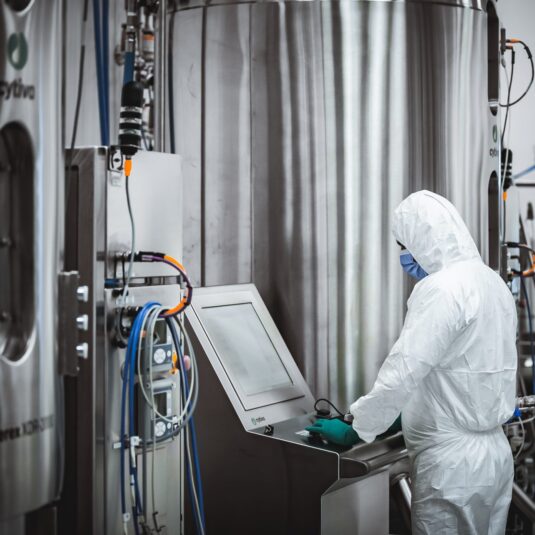
Navigating OOX Results: Effective Analysis and Management in CDMO Laboratories
Analytics, Manufacturing, Regulatory
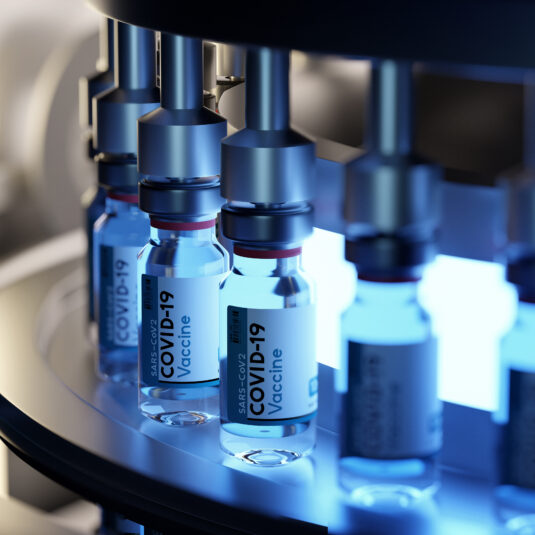
Mammalian cell lines as a platform for protein vaccine production
Cell culture, Drug substance, Manufacturing, Proteins, Vaccines